Tim Fister
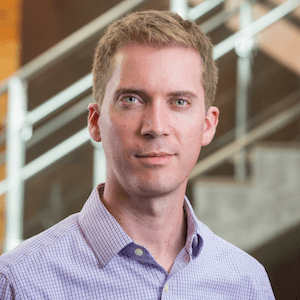
Bio
Tim Fister is a principal investigator on multiple DOE and Industry-led projects related to energy storage, with nearly ten years’ experience working with the lead battery manufacturers. His work centers on the real-time characterization of battery materials during synthesis and cycling using synchrotron x-ray scattering, spectroscopy, and imaging methods, which has resulted in over one hundred publications.
Tim Fister,1 Tiffany Kinnibrugh,2 Kevin Knehr,1 Mohammed Effat,1 Jae Jin Kim,1 Seongjun Kim,2 Juan Garcia,1 Hakim Iddir,1 Julian Kosacki,3 Matthew Spence3
- Argonne National Laboratory, Chemical Sciences and Engineering Division
- Argonne National Laboratory, X-ray Science Division
- Clarios
Emerging markets in long duration energy storage and auxiliary batteries for electric vehicles will require lead batteries capable of deep discharge over hundreds to thousands of cycles. The most common failure mode in these applications is related to the positive active material (PAM), where maintaining interparticle connectivity and connectivity to the positive grid becomes increasingly challenging. Deep-cycle batteries often rely on a high αPbO2 content after formation, but gradually transition to PAM with predominantly βPbO2 during cycling. Here, we demonstrate the conditions that promote α and β PbO2 during formation, as well as the surprising presence of residual PbO in commercial battery plates formed with varying acid concentration, current density, soak time, and temperature. These conditions also apply to the substantial swings possible in positive electrodes during cycling that can approach neutral or even locally alkaline conditions at deep discharge. Within this dataset, we follow changes in PAM composition and particle size during initial cycling and at end-of-life, where the electrodes are nearly 100% βPbO2 with crystallites that have ripened ten-fold in size than after formation. Using both atomic-scale and continuum modeling combined with high-resolution 3D X-ray imaging, we provide mechanisms explaining this evolution and models for extending cycle life at deep discharge.